The research, published July 6 in the Proceedings of the National Academy of Science of the United States of America (PNAS), was conducted by a team of scientists under the direction of Prof. Prof. Weilin Xu of the Changchun Institute of Applied Chemistry, CAS, and Prof. A. Paul Alivisatos of the University of California at Berkeley, using the newly developed technique of optical super-resolution imaging. The study reveals great progress in understanding how nanocatalysts work spatiotemporally at the level of a single molecule or particle.
Nanomaterials are now used extensively in everyday life and nanocatalysts are one member of this category. Nanocatalysts play a key role in the activity of fuel cells, which are used to power environmentally friendly fuel cell vehicles that run on hydrogen and generate no emissions. Nanocatalysts in such cells speed up the transformation to electricity of chemical energy from fuel .
To some extent, however, fuel cell vehicles are still a "concept" vehicle. They showcase new technology but are too costly to be broadly popular. Most of the high cost comes from the use of precious metals (such as platinum, Pt) in fuel cells as electrocatalysts. For this reason, research is needed to understand how nanocatalysts work. Such research could reduce the consumption of precious metals by designing more efficient precious metal-based catalysts or finding other cheap alternatives for platinum.
Tremendous efforts have been made in the past several decades by scientists around the world to understand nanocatalysts. It is now known that the facets, defects or corners of a nanocatalyst can have very distinct "static" catalytic activity. But how spatiotemporally or “dynamically” different are the catalytic properties of these parts?
The study in PNAS, employed time-lapse super-resolution mapping of single-molecule catalysis events on individual nanoparticles to observe changes over time in the spatial distribution of catalysis events on individual nanocatalysts. Compared with the active sites on well-defined surface facets, the defects on the nanocatalysts were found to possess higher intrinsic reactivity but lower stability. Corners and ends were more reactive but also less stable than flat surfaces. On average, the most stable sites dominate the total apparent activity of single nanocatalysts. However, for the first time, researchers found that active sites with higher intrinsic activity but lower stability showed activity at an earlier time before deactivating. Interestingly, the “self-healing” of some active sites was observed after deactivation for a while, probably due to desorption of the adsorbate.
The results obtained in this work through spatiotemporal super-resolution measurement of different types of active catalytic sites on single particles will lead to a more comprehensive understanding of reactivity patterns and may enable the rational design of new and more productive heterogeneous catalysts, such as precious metal-based electrocatalysts used in fuel cell vehicles.
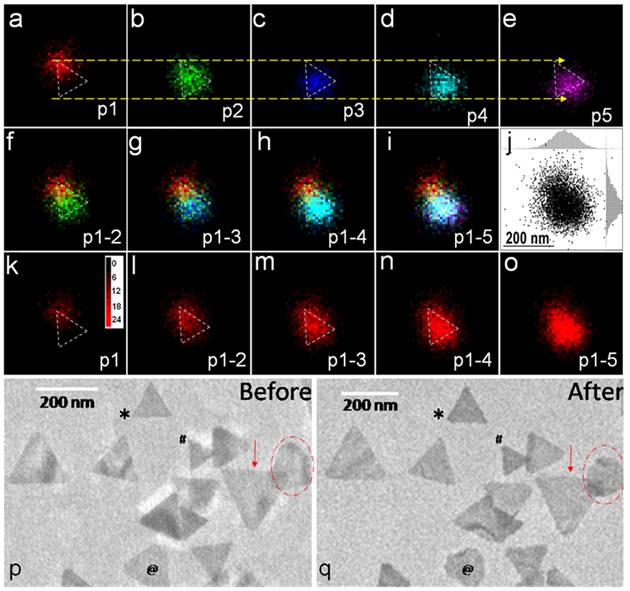
Fig. (a-o) Two-dimensional time-lapse single-molecule nanocatalysis mapping on a single Au nanoplate. (p-q)The morphology of the same set of Au nanoplates studied with TEM before (p)/after (q) the fluorogenic reaction. The symbols mark the variations of corners and shapes of Au nanoplates after reaction.